Work and power in straight-line motion efficiency. Technical mechanics. Conservation laws. Complex tasks
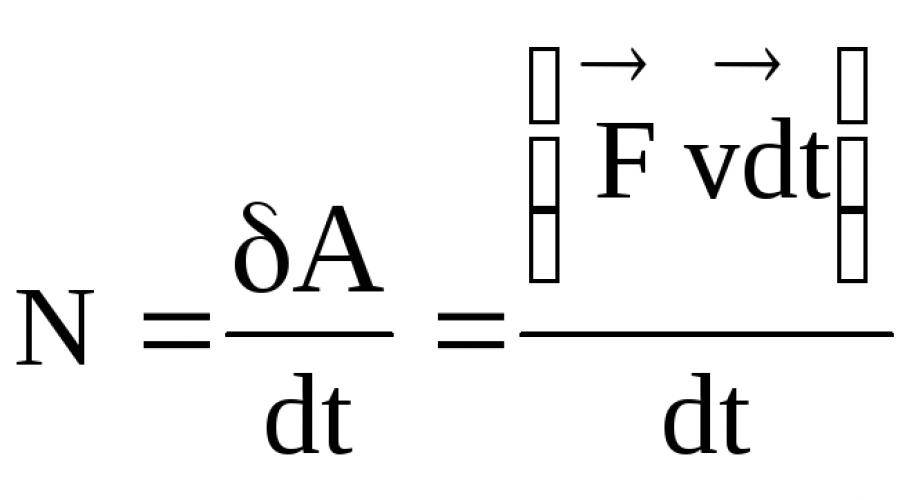
Electric motors have a high coefficient of performance (efficiency), but it is still far from the ideal indicators that designers continue to strive for. The thing is that during the operation of the power unit, the conversion of one type of energy into another takes place with the release of heat and inevitable losses. The dissipation of thermal energy can be recorded in different components of any type of engine. Power losses in electric motors are a consequence of local losses in the winding, in steel parts and during mechanical operation. Additional losses contribute, albeit insignificantly.
Magnetic power loss
When magnetization reversal occurs in the magnetic field of the armature core of an electric motor, magnetic losses occur. Their value, consisting of the total losses of eddy currents and those that arise during magnetization reversal, depends on the frequency of magnetization reversal, the values of the magnetic induction of the back and armature teeth. A significant role is played by the thickness of the sheets of electrical steel used and the quality of its insulation.
Mechanical and electrical losses
Mechanical losses during operation of an electric motor, like magnetic ones, are permanent. They consist of losses due to bearing friction, brush friction, and engine ventilation. The use of modern materials, the performance characteristics of which are improving from year to year, allows minimizing mechanical losses. In contrast, electrical losses are not constant and depend on the load level of the electric motor. Most often they arise due to heating of brushes and brush contact. The efficiency decreases due to losses in the armature winding and excitation circuit. Mechanical and electrical losses are the main contributors to changes in engine efficiency.
Additional losses
Additional power losses in electric motors consist of losses arising in equalizing connections and losses due to uneven induction in the armature steel at high loads. Eddy currents, as well as losses in pole pieces, contribute to the total amount of additional losses. It is quite difficult to accurately determine all these values, so their sum is usually taken to be within the range of 0.5-1%. These figures are used to calculate the total losses to determine the efficiency of the electric motor.
Efficiency and its dependence on load
The coefficient of performance (COP) of an electric motor is the ratio of the useful power of the power unit to the power consumed. This indicator for engines with a power of up to 100 kW ranges from 0.75 to 0.9. for more powerful power units, the efficiency is significantly higher: 0.9-0.97. By determining the total power losses in electric motors, the efficiency of any power unit can be calculated quite accurately. This method of determining efficiency is called indirect and it can be used for machines of various powers. For low-power power units, the direct load method is often used, which consists of measuring the power consumed by the engine.
The efficiency of an electric motor is not a constant value; it reaches its maximum at loads of about 80% of power. It reaches its peak value quickly and confidently, but after its maximum it begins to slowly decrease. This is associated with an increase in electrical losses at loads exceeding 80% of the rated power. The drop in efficiency is not large, which suggests high efficiency indicators of electric motors over a wide power range.
It is known that a perpetual motion machine is impossible. This is due to the fact that for any mechanism the following statement is true: the total work done with the help of this mechanism (including heating the mechanism and the environment, overcoming the friction force) is always greater than the useful work.
For example, more than half of the work of an internal combustion engine is wasted on heating the engine components; some heat is carried away by the exhaust gases.
It is often necessary to evaluate the effectiveness of the mechanism and the feasibility of its use. Therefore, in order to calculate what part of the work done is wasted and what part is useful, a special physical quantity is introduced that shows the efficiency of the mechanism.
This value is called the efficiency of the mechanism
The efficiency of a mechanism is equal to the ratio of useful work to total work. Obviously, the efficiency is always less than one. This value is often expressed as a percentage. It is usually denoted by the Greek letter η (read “this”). The efficiency factor is abbreviated as efficiency.
η = (A_full /A_useful) * 100%,
where η efficiency, A_full total work, A_useful useful work.
Among engines, the electric motor has the highest efficiency (up to 98%). The efficiency of internal combustion engines is 20% - 40%, and that of a steam turbine is approximately 30%.
Note that for increasing the efficiency of the mechanism often try to reduce the friction force. This can be done using various lubricants or ball bearings in which sliding friction is replaced by rolling friction.
Examples of efficiency calculations
Let's look at an example. A cyclist weighing 55 kg rode a bicycle weighing 5 kg up a hill 10 m high, doing 8 kJ of work. Find the efficiency of the bicycle. Do not take into account the rolling friction of the wheels on the road.
Solution. Let's find the total mass of the bicycle and the cyclist:
m = 55 kg + 5 kg = 60 kg
Let's find their total weight:
P = mg = 60 kg * 10 N/kg = 600 N
Let's find the work done to lift the bicycle and the cyclist:
Auseful = PS = 600 N * 10 m = 6 kJ
Let's find the efficiency of the bicycle:
A_full / A_useful * 100% = 6 kJ / 8 kJ * 100% = 75%
Answer: The efficiency of the bicycle is 75%.
Let's look at another example. A body of mass m is suspended from the end of the lever arm. A downward force F is applied to the other arm, and its end is lowered by h. Find how much the body rose if the efficiency of the lever is η%.
Solution. Let's find the work done by force F:
η% of this work is done to lift a body of mass m. Consequently, Fhη / 100 was spent on raising the body. Since the weight of the body is equal to mg, the body rose to a height of Fhη / 100 / mg.
In practice, it is important to know how quickly a machine or mechanism does work.
The speed at which work is done is characterized by power.
Average power is numerically equal to the ratio of work to the period of time during which work is performed.
If Dt ® 0, then, going to the limit, we obtain the instantaneous power:
.
(8)
,
(9)
N = Fvcos.
In SI, power is measured in watts(Wt).
In practice, it is important to know the performance of mechanisms and machines or other industrial and agricultural equipment.
For this purpose, the coefficient of performance (efficiency) is used.
The efficiency factor is the ratio of useful work to all expended.
.
(10)
.
1.5. Kinetic energy
The energy possessed by moving bodies is called kinetic energy(W k).
Let's find the total work done by the force when moving the m.t. (body) along the path section 1–2. Under the influence of force, the m.t. can change its speed, for example, it increases (decreases) from v 1 to v 2.
We write the equation of motion of m.T. in the form
Full work or
.
After integration ,
Where called kinetic energy. (eleven)
Therefore,
.
(12)
Conclusion: The work done by a force when moving a material point is equal to the change in its kinetic energy.
The obtained result can be generalized to the case of an arbitrary m.t. system: .
Consequently, the total kinetic energy is an additive quantity. Another form of writing the kinetic energy formula is widely used: .
(13)
Comment: kinetic energy is a function of the state of the system, depends on the choice of reference system and is a relative quantity.
In the formula A 12 = W k, A 12 must be understood as the work of all external and internal forces. But the sum of all internal forces is zero (based on Newton's third law) and the total momentum is zero.
But this is not the case in the case of the kinetic energy of an isolated system of m.t. or bodies. It turns out that the work done by all internal forces is not zero.
As can be seen from Fig. 6, the work done by force f 12 to move a m.t. with mass m 1 is positive
A 12 = (– f 12) (– r 12) > 0
and the work of force f 21 to move m.t. (body) with mass m 2 is also positive:
A 21 = (+ f 21) (+ r 21) > 0.
Consequently, the total work of the internal forces of an isolated m.t. system is not equal to zero:
A = A 12 + A 21 0.
Thus, the total work of all internal and external forces goes to change kinetic energy.
Power is inherently the speed at which work is done. The greater the power of the work performed, the more work is performed per unit of time.
Average power is the work done per unit of time.
The amount of power is directly proportional to the amount of work done \( A\) and inversely proportional to time \( t\) for which the work was completed.
Power\( N\) determined by the formula:
The unit of measurement of power in the \(SI\) system is \(Watt\) (Russian designation - \(W\), international - \(W\)).
To determine the engine power of cars and other vehicles, a historically more ancient unit of measurement is used - Horsepower (hp), 1 hp = 736 W.
Example:
The car engine power is approximately \(90 hp = 66240 W\).
The power of a car or other vehicle can be calculated if the traction force of the car is known \( F\) and the speed of its movement ( v).
This formula is obtained by transforming the basic formula for determining power.
Not a single device is capable of using \(100\)% of the energy initially supplied to it to perform useful work. Therefore, an important characteristic of any device is not only power, but also efficiency , which shows how efficiently the energy supplied to the device is used.
Example:
In order for a car to move, the wheels must rotate. And in order for the wheels to rotate, the engine must drive the crank mechanism (the mechanism that converts the reciprocating movement of the engine piston into rotational movement of the wheels). In this case, the gears are driven into rotation and most of the energy is released in the form of heat into the surrounding space, resulting in a loss of supplied energy. The efficiency of a car engine is within \(40 - 45\)%. Thus, it turns out that only about \(40\)% of the total gasoline that is used to fill the car goes to perform the useful work we need - moving the car.
If we fill the car's tank with \(20\) liters of gasoline, then only \(8\) liters will be spent on moving the car, and \(12\) liters will burn without doing any useful work.
The efficiency factor is denoted by the letter of the Greek alphabet \("eta"\) η, it is the ratio of the useful power \( N\) to the total or total power N total.
To determine it, use the formula: η = N N total. Since, by definition, efficiency is a power ratio, it has no unit of measurement.
It is often expressed as a percentage. If the efficiency is expressed as a percentage, then use the formula: η = N N total ⋅ 100%.
Job A
– a scalar physical quantity measured by the product of the modulus of the force acting on the body, the modulus of its displacement under the influence of this force and the cosine of the angle between the vectors of force and displacement:
Module of body movement, under the influence of force,
The work done by the force
On graphs in axes F-S(Fig. 1) the work of force is numerically equal to the area of the figure limited by the graph, the axis of displacement and straight lines parallel to the axis of force.
If several forces act on a body, then in the work formula F- this is not the resultant ma of all these forces, but precisely the force that does the work. If a locomotive pulls cars, then this force is the traction force of the locomotive; if a body is lifted on a rope, then this force is the tension force of the rope. This can be both the force of gravity and the force of friction, if the problem statement deals with the work of these particular forces.
Example 1. A body weighing 2 kg under the influence of force F moves up an inclined plane at a distance. The distance of the body from the Earth's surface increases by .
Force vector F directed parallel to the inclined plane, force modulus F is equal to 30 N. What work was done by the force during this movement in the reference frame associated with the inclined plane F? Take the free fall acceleration equal to , friction coefficient
Solution: The work of a force is defined as the scalar product of the force vector and the displacement vector of the body. Therefore, the strength F performed work when lifting a body up an inclined plane.
If the problem statement talks about the coefficient of performance (COP) of any mechanism, you need to think about what kind of work it does is useful and what kind of work is wasted.
Mechanism efficiency factor (efficiency) η They call the ratio of useful work done by a mechanism to all the work expended.
Useful work is that which needs to be done, and spent work is that which actually has to be done.
Example 2. Let a body of mass m be raised to a height h, moving it along an inclined plane of length l under the influence of traction F thrust. In this case, the useful work is equal to the product of gravity and the lifting height:
And the work expended will be equal to the product of the traction force and the length of the inclined plane:
This means that the efficiency of the inclined plane is:
Comment: The efficiency of any mechanism cannot be more than 100% - the golden rule of mechanics.
Power N (W) is a quantitative measure of the speed of work. Power is equal to the ratio of work to the time during which it was completed:
Power is a scalar quantity.
If the body moves uniformly, then we get:
Where is the speed of uniform motion.